NASA/JPL-Caltech/ O. Krause (Steward Observatory)
Ever since neutron stars had been found, researchers have been utilizing their uncommon properties to probe our universe. The superdense remnants of stellar explosions, neutron stars pack a mass larger than the Sun’s into a ball about as broad as San Francisco. A single cup of this star matter would weigh about as a lot as Mount Everest.
These odd celestial our bodies may alert us to distant disturbances in the material of spacetime, educate us about the formation of components, and unlock the secrets and techniques of how gravity and particle physics work in some of the most excessive situations in the universe.
“They’re at the center of a lot of open questions in astronomy and astrophysics,” says astrophysicist Vanessa Graber of the Institute of Space Sciences in Barcelona.
But to precisely interpret some of the neutron stars’ indicators, researchers should first perceive what goes on inside them. They have their hunches, however experimenting straight on a neutron star is out of the query. So scientists want one other option to take a look at their theories. The conduct of matter in such a superdense object is so difficult that even laptop simulations aren’t as much as the process. But researchers suppose they could have discovered a resolution: an earthly analog.
Though younger neutron stars can have temperatures in the tens of millions of levels of their inside, by one essential energetic measure neutrons are thought of “cold.” Physicists suppose that’s a attribute they’ll exploit to review the interior workings of neutron stars. Instead of trying to the sky, researchers are peering into clouds of ultracold atoms created in laboratories right here on Earth. And that may assist them lastly reply some longstanding questions on these enigmatic objects.
Space oddities
The existence of neutron stars was first proposed in 1934, two years after the discovery of the neutron itself, when astronomers Walter Baade and Fritz Zwicky puzzled if a celestial physique made completely of neutrons may stay after a supernova explosion. Though they didn’t get all the particulars proper, their basic thought is now broadly accepted.
Stars energy themselves by fusing the nuclei of lighter atoms into these of heavier atoms. But when stars run out of these lighter atoms, nuclear fusion stops and there’s no longer an outward strain to combat towards the inward power of gravity. The core collapses and the star’s outer layer races inward. When this layer hits the dense core, it bounces off and explodes outward, producing a supernova. The dense core that continues to be afterward is a neutron star.
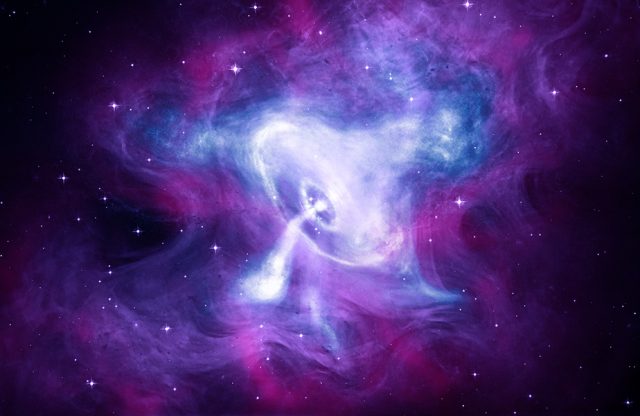
CREDIT: NASA: X-RAY: CHANDRA (CXC), OPTICAL: HUBBLE (STSCI), INFRARED: SPITZER (JPL-CALTECH)
It wasn’t till the Sixties that Zwicky and Baade’s hypothetical neutron stars had been lastly detected. Radio astronomer Jocelyn Bell Burnell seen a unusual, commonly pulsed radio wave sign from area whereas working as a graduate scholar at the University of Cambridge. She was detecting one thing that had by no means been seen earlier than: a particular type of neutron star known as a pulsar, which flashes beams of radiation at common intervals because it spins, like a lighthouse. (Her adviser, alongside with the director of the observatory—however not Bell Burnell—later acquired the Nobel Prize for the discovery.)
Since then, 1000’s of neutron stars have been detected. As some of the densest, highest-pressure objects in the universe, neutron stars may assist us study what occurs to matter at extraordinarily excessive densities. Understanding their construction and the conduct of the neutron matter composing them is of paramount significance to physicists.
Scientists already know that the neutrons, protons, and different subatomic particles that compose a neutron star organize themselves in another way relying on the place in the star they’re. In sure sections, they pack rigidly like water molecules in a block of ice. In others, they circulation and swirl like a frictionless fluid. But precisely the place the transition occurs and the way the totally different phases of matter behave, physicists aren’t certain.
A superdense star born of a nuclear fireball appears, on its face, to have little or no in frequent with a dilute cloud of ultracold particles. But they’ll share no less than one helpful attribute: They are each beneath a threshold generally known as the Fermi temperature that depends upon—and is calculated primarily based on—the matter every system is made of. A system that’s effectively above this temperature will largely behave based on the legal guidelines of classical physics; whether it is effectively beneath, its conduct will probably be dominated by quantum mechanics. Certain ultracold gases and neutron star materials can each be effectively beneath their Fermi temperatures and consequently can act in comparable methods, says Christopher Pethick, a theoretical physicist at the Niels Bohr Institute in Copenhagen and coauthor of an early overview of neutron stars in the 1975 Annual Review of Nuclear Science.
Matter that’s beneath its Fermi temperature can obey remarkably common legal guidelines. This universality signifies that, whereas we don’t have quick access to several-million-degree neutron star matter, we may study some of its conduct by experimenting with ultracold gases that may be created and manipulated in laboratory vacuum chambers on Earth, says theoretical astrophysicist James Lattimer of Stony Brook University in New York, writer of a abstract of the science of nuclear matter in the 2012 Annual Review of Nuclear and Particle Science.
Of specific curiosity to Lattimer is a theoretical state known as a unitary gasoline. A gasoline is unitary when every of its particles’ sphere of affect turns into infinite, that means that they’d affect one another regardless of how far aside they’re. This is inconceivable to have in actuality, however ultracold atom clouds can get shut—and so can the matter inside of neutron stars. “It’s similar to a unitary gas,” Lattimer says, “but it’s not a perfect unitary gas.”